Related policy objectives
Overview
Integrated agricultural production systems are mixed systems in which by-products of one component of the system serve as resources for another component. In integrated crop-livestock systems (ICLS), crop and livestock production are mutually supportive and mutually dependent. ICLS are distinct from specialized systems, where crops and livestock production are decoupled and depend on external inputs. ICLS close the loop in nutrient and energy cycles. For example, livestock transform plant residues and by-products into edible high-quality protein and manure, which is used as an organic fertilizer, replacing dependency on mineral fertilizers. Re-coupling crop and livestock systems at farm and/or territorial scales can help to reduce environmental externalities associated with conventional commercial farming(e.g. GHG emissions from production of mineral fertilizers) and increase the resilience of smallholder farmers (e.g. by diversifying income and diet). As integrated systems are diverse, at the landscape level, they can also be developed in a range of habitats and enhance agricultural biodiversity.
Concrete measures to implement
To manage ICLS at the farm scale, FAO suggests farmers focusing on the following measures:
- Improve cycling and reduce losses of nutrients:
- Use animal manure and slurry for organic fertilization of crops and trees, reducing dependency on externally sourced fertilizers. Note that the amount and timing of manure application should be carefully planned to avoid detrimental impacts on environment and groundwater. (See Reducing emissions from livestock through sustainable management practices.
- Feed livestock using crop residues (e.g. weeds, straw, stover stubble, green regrowth of crop or fallen grain), and crop processing by-products (e.g. bran, molasses or pulps). Note that it is important to balance the use of crop residue for animal feed and for soil cover.
- Cover soil with “catch crops,” which are secondary crops grown in the time interval between two main crops or planted between the rows of a main crop. Catch crops can retain potentially leached resources (e.g. nutrients and water), prevent erosion, improve soil fertility and also be used for animal feed or mulch.
- Take all farm system demands into account, including the needs for animal feed. Instead of selecting crop varieties only for higher yield, use varieties that have, for example, better quality straws, more straws or higher ratooning rates (i.e., post-harvest regrowth of the crop).
- Improve cropping patterns:
- Implement integrated grazing management, which involves rotation of annual crops and pasture, and when possible, leaving land fallow to help restore soil fertility.
- Use leys. A ley is a field in which crops are grown in rotation with grass for pasture or legumes. The mix of rotational cropping and organic fertilization through livestock accelerates soil fertility restoration.
- Integrate companion cropping to improve soil quality and yields. For example, one companion crop combination could involve tropical forage grasses combined with cash crops like coffee, citrus, or soy.
- Integrate cereal-legume intercropping into crop rotations, which provide staple cereal yields and high-quality forage for livestock (e.g., alfalfa or cowpeas with oats).
- Implement landscape mosaics where different areas are reserved for different purposes, including crops for human consumption, forage crops and grassland. Combining grass and arable cropping lands is “an essential foundation” for integrated crop-livestock systems.
- Integrate a greater variety of crops,animals, and plants that can potentially provide transformative market opportunities for farmers.
- Use livestock for energy provision: Switch from unsustainable fuel sources (e.g. wood, charcoal, kerosene or oil) to manure-based biogas or dung cakes for powering household (e.g. cooking and lighting) or rural-industry (e.g. mills or water pumps) activities.
- Establish sustainable Integrated-Agriculture-Aquaculture (IAA) systems (e.g., rice-fish cultivation systems) to offer small-scale producers in rural areas greater income diversification and nutrition. See guidance on Implementing sustainable aquaculture management systems.
Enabling governance measures
ICLS face barriers that limit their adoption. To support farmers transitioning to ICLS, governments can:
- Support local communities, smallholder farmers, social movements and marginalised groups that seek self-sufficiency and/or promote holistic and agroecological movements, which include reducing reliance on external inputs. Adopt equitable, participatory land management and governance for more long-term ICLS at landscape scale.
- Increase research investments in sustainable livestock production technologies and management that are tailored to local conditions.
- Support transformative, equitable market opportunities from the integration of crop rotations that produce additional marketable crops.
- Provide access to finance, especially to de-risk initial investments, as lack of access to finance is one of the major barriers to ICLS uptake.
- Provide subsidies to reward sustainable practices, such as crop diversification. Examples include the European Common Agricultural Policy (CAP) subsidy to maintain grasslands and seminatural areas; Brazil’s ABC programme that subsidized loans for ICLS adoption; and New Zealand’s policies to improve nutrient management.
- Provide appropriate education, training and capacity-building for agricultural producers and extension officers to recognize the benefits of ICLS for food security.
- Secure clear tenure and resource rights, especially for smallholders, women, Indigenous Peoples and Local Communities. Land managers and farmers are more likely to invest in soil management measures if their land rights are sufficient and secure.
- Improve equitable access to resources such as markets for inputs, outputs, and financial services, or government support for smallholders, women, Indigenous Peoples, Local Communities, youth and other disenfranchised groups.
- Invest in the expansion of decent rural farm employment, non-farm employment, and livelihood opportunities, especially for women and youth, by investing in entrepreneurship, small and medium enterprises, smallholder, and family operations to ensure equitable, inclusive, and decent opportunities for income.
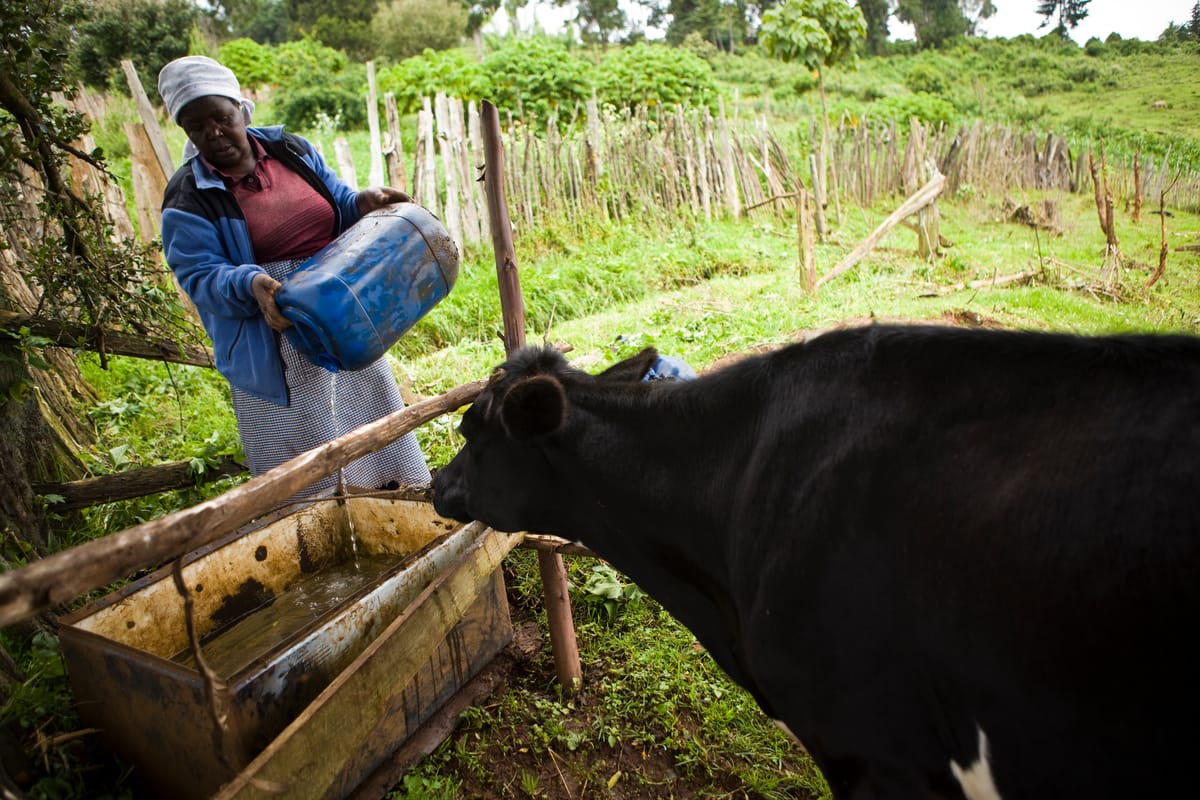
Tools and MRV systems to monitor progress
GLEAM-i
An open-source tool to calculate GHG emissions from livestock production.
The EX-Ante Carbon-balance Tool (EX-ACT)
A tool to estimate and track the outcomes of agricultural interventions on GHG emissions.
The EX-Ante Carbon-balance Tool
For value chains (EX-ACT VC) is a tool to calculate GHG emissions along agri-food value chains.
FAO Climate Smart Agriculture Sourcebook
FAO provides an open-source, online Climate Smart Agriculture Sourcebook that contains a module on climate-smart integrated production systems.
Practical guide on ecological soil management
The 2021 edition of the practical guide on ecological soil management by the Sustainable Agriculture Research and Education programme at the University of Maryland, US, contains a chapter on integrating crops and livestock.
AgSTAR’s Anaerobic Digester Project Development Handbook
Compiles best practices for anaerobic digestion and biogas systems which can be a part of integrated crop-livestock systems.
Collect Earth Online
A free, open-source system developed by NASA and USAID and hosted by FAO for viewing and interpreting high-resolution satellite imagery. Users can collect up-to-date information or conduct assessments related to land cover, land use, forestry and agriculture.
Climate Risk Planning & Managing Tool for Development Programmes in Agri-Food Systems (CRISP)
An interactive tool supporting the mainstreaming of climate adaptation options, including recommendations on crop-livestock management, for agricultural farming systems.
GrassSignal
A decision support tool for sustainable grassland monitoring.
Piipponen et al. (2022)
Provides data to estimate grasslands livestock carrying capacity.
Mitigation benefits
- The emission intensity of integrated production systems is typically lower than that of specialized production systems. Implementation of integrated production systems lowers overall GHG emissions from agriculture.
- Integrated crop-livestock systems could reduce enteric methane emissions by up to 17% in OECD countries, 24% in East Africa and 38% in South Asia.
- LCLS contribute to climate change mitigation in multiple ways:
- Using manure for crop production avoids GHG emissions from the production, transport and application of synthetic fertilizers.
- Applying manure inputs biomass to the soil, which increases soil organic matter and thus soil carbon sequestration.
- Using crop residues, by-products and locally produced forage for livestock feed avoids emissions from the disposal of residues and feed transportation. It can also help to reduce the amount of land area needed for production of feed crops and thereby avoids GHG emissions related to land-use change, which FAO estimates is the largest source of emissions from global livestock production, accounting for 45% of total livestock production emissions (including emissions from land-use change).
- Feeding more digestible feed (e.g. pasture, crop residues, fodder trees and shrubs) to livestock such as ruminants, pigs and poultry improves the dietary quality for animals and lowers methane emissions from enteric fermentation and manure.
- Feeding crop residues and by-products to livestock alleviates pressure on grasslands and increases grassland restoration and quality, which increases grasslands’ ability to take up and store carbon.
- Growing perennial forages instead of annual cash crops increases soil carbon sequestration by extending the growing season, which minimizes soil disturbance and associated carbon emissions.
- Overall, the rates of carbon sequestration in the soil attain a maximum level in 5-20 years after adoption of integrated management practices. After this, carbon sequestration continues but sequestration rates decrease until soil organic carbon stocks reach a saturation point, which is determined mainly by soil texture and the chemical composition of soil organic matter. There is great potential for improving soil carbon stocks in eroded and degraded soils.
Other environmental benefits
- Potential reduction of air pollution, through switch to cleaner fuel sources.
- Increased water infiltration into soil, through enhanced soil cover and soil organic matter content.
Adaptation benefits
See nature-positive crop production, sustainable livestock production, agroforestry, soil carbon management, and improved management of grasslands and pastures highlight further benefits of some techniques that are also used in integrated crop-livestock systems. The following are some of the main adaptation benefits of ICLS:
- Resilience and risk management: increased resilience of farm-level production to adverse climatic and economic events. Greater flexibility of production systems in coping with potential socio-economic and climate change-induced hazards and crises, as producers have a wider range of risk management strategies and response options.
- Reduced soil erosion and enhanced soil fertilization, soil productivity and nutrient cycling.
- Protection against extreme weather and pests: improved control of pests and diseases, improved weed control and protection against climate-related stress.
- Livelihoods: improved farm productivity resulting in diversified and increased farm income.
- Increased water infiltration into soil.
Other sustainable development benefits
- SDG 1 (No poverty) and SDG 8 (Decent work and economic growth): ICLS increase farm-level profitability by reducing operating costs associated with inputs, pollution and waste. At the same time, integrated systems increase farm-level productivity and income as well as economic resilience of livelihoods. For example, in soy production, integrated system productivity greatly exceeds specialized system productivity. All these factors reduce poverty and promote sustainable economic growth at the household level.
- SDG 2 (Zero hunger) & SDG 3 (Good health and well-being): ICLS improve food security and nutrition and promote health and well-being by increasing dietary diversity and its consequent health benefits.
- SDG 2 (Zero hunger) & SDG 12 (Responsible consumption and production): ICLS promote sustainable agriculture and ensure sustainable production patterns. Intensified farm-level cycling of nutrients, water and energy minimizes the environmental impacts of production. Improved efficiency in carbon and nitrogen cycles contributes to sustainable production intensification and diversification.
- SDG 15 (Life on land): ICLS protect and promote the sustainable use of terrestrial ecosystems through:
- Increased landscape and habitat diversity, which benefit food chains within ecosystems and increase interactions between groups of organisms.
- Increased conservation of farm-level agricultural biodiversity.
- Diversified and increased ecosystem services, including water quality preservation.
- Decreased ambient pollution from livestock production compared to feedlot-based production.
- Improved animal health through avoidance of parasitism, emergent diseases and improved dietary quality.
- Increased seed dispersal, plant diversity and habitat provision, especially for pollinators, through integration of pastures and crops.
Main implementation challenges, potential negative externalities and trade-offs
- Implementation of integrated production systems requires substantial knowledge, access to technical support (e.g. extension services), and potentially high upfront investments (e.g. costs of equipment needed for energy production from agricultural by-products).
- Poor access to markets, insurance and credit undermines the economic viability of ICLS.
- Financial incentive systems favour specialized production systems (e.g. through subsidization of inputs or lack of subsidies for integrated production systems).
- ICLS can be vulnerable to disturbance because the mixing of resource flows between system components makes the systems internally more complex and interdependent. ICLS become more vulnerable to disturbance if individual system components area highly sensitive to ecological change.
- CH4 emissions from cattle and N2O emissions from crops and pastures may neutralize the benefits of soil organic carbon storage but the precise effects are unclear and require more research.
- Trade-offs between using crop residues to feed livestock versus to improve soil health need to be balanced. Depending on the location and level of degradation, arable parts of farm systems may need to retain crop residues to improve soil health and will therefore not be able to reduce grazing pressure on grasslands or feed external sourcing.
- Environmental benefits of grassland ecosystems can be impaired as intensification of livestock production increases. Increased livestock production risks overgrazing and soil compaction through trampling.
- Productivity of individual system components in ICLS may be lower than in specialized production systems even though overall productivity of integrated systems greatly exceeds those of specialized systems. For example, average soybean yields and aboveground cover crop biomass production are lower in integrated systems than in specialized soy production systems but additional forage and livestock production increases total system output.
Measures to minimise challenges, potential negative externalities and trade-offs
- Adequate provision and adoption of appropriate inputs, supplies, technologies and management practices for each system component.
- Capacity building for producers as well as for agricultural extension officers on manure management, compost/organic fertilizer production and optimization, and catch/cover crops.
- Strengthening inclusive and equitable local institutions.
- Establish inclusive and equitable producer organisations.
- Mainstreaming of integrated production systems into curricula of agricultural education institutions.
- Financial risk assessment and finance plan in planning phase.
- Provision of dedicated credit lines and/or financial support/incentives including payment for environmental services.
- Appropriate selection of crop and livestock species and breed
- Rotational grazing, as opposed to continuous grazing, allows the vegetation to recover between grazing events.
- Feeding animals indoors with hay or silage harvest of forage can help to balance grasslands utilization and environmental carrying capacity.
- Management plans to control herd density and grazing time avoid soil compaction and overgrazing from livestock production and optimize vegetation and livestock rotation.
- Overgrazing during specific periods of the season, such as extended dry periods where grasslands can be vulnerable to lasting damage, can be managed through zero-grazing methods, whereby herders keep their animals in stables, and ‘cut-and-carry’ biomass to feed them. While this is not recommended for long periods of time, it can ensure overgrazing does not occur during critical periods of the season.
- FAO recommends adopting a holistic perspective on ICLS integrated crop-livestock systems where the different system components act as one entity. Therefore, achieving a high yield for the combination of the components should be the focus rather than achieving a high yield for one component only.
Implementation costs
- Research conducted in Mato Grosso, Brazil, from 2005-12 shows that ICLS require an initial investment of USD 863/ha. This is higher than the initial investment costs for traditional specialized livestock systems (USD 174/ha) or specialized soybean or corn production (USD 766/ha). For ICLS including cattle and either soy or corn, the operational costs of a typical farm ranged from USD 110-283/ha and the input costs from USD 80-181/ha. This is lower than the operational and input costs of specialized soy and corn farms (total operational and input costs USD 860-1,484/ha) but higher than those of specialized cattle farms.
Intervention in practice
- Between 2011 and 2014, the FAO Mitigation of Climate Change Programme (MICCA), which was designed by FAO with financial support from the Government of Finland to research and expand climate-smart agriculture, implemented a pilot project on integrated crop-livestock-tree farming systems in Kaptumo, Kenya. The project aimed to reduce the overall GHG balance of livestock production systems in the area and was realized by the Kaptumo Dairy Farmer Business Association (DFBA) within the East Africa Dairy Development (EADD) Programme. Through an innovative farmer-to-farmer extension approach, about 4,700 smallholder farmers were trained on improved on-farm fodder production and conservation, improved manure and gazing management, and awareness and coping capacity regarding climate change. Farmers were also given loans to facilitate implementation of practices such as manure collection, composting, bush clearing, paddocking or spot and strip sowing with legumes. Participating farmers reported higher yields, raised farm income and increased food availability.
References/Bibliography
- Abagandura, G. O., Şentürklü, S., Singh, N., Kumar, S., Landblom, D. G., & Ringwall, K. (2019). Impacts of crop rotational diversity and grazing under integrated crop-livestock system on soil surface greenhouse gas fluxes. PLoS ONE, 14(5). Retrieved February 6, 2024, from https://www.ncbi.nlm.nih.gov/pmc/articles/PMC6530893/
- Aguilar, J., Archer, D., Hendrickson, J., Kronberg, S., Liebig, M., Nichols, K., et al. (2013). Diversification and ecosystem services for conservation agriculture: Outcomes from pastures and integrated crop–livestock systems. Renewable Agriculture and Food Systems, 28(2), 129–144.
- Chen, R. F. F., Garrett, R. D., Kamoi, M. Y. T., Latorraca, D., Michetti, M., Rodrigues, R. de A. R., et al. (2020). Assessing the economic viability of integrated crop−livestock systems in Mato Grosso, Brazil. Renewable Agriculture and Food Systems, 35(6), 631–642.
- Climate Smart Agriculture Sourcebook. (n.d.). Retrieved February 6, 2024, from https://www.fao.org/climate-smart-agriculture-sourcebook/production-resources/module-b1-crops/b1-overview/en/
- Cruz Colazo, J., de Dios Herrero, J., Sager, R., Guzmán, M. L., & Zaman, M. (2022). Contribution of Integrated Crop Livestock Systems to Climate Smart Agriculture in Argentina. Land, 11(11), 2060.
- FAO. (2001). Mixed crop-livestock farming: A review of traditional technologies based on literature and field experience. Retrieved from https://www.fao.org/3/Y0501E/Y0501E00.htm.
- FAO. (2016). Planning, implementing, and evaluating Climate-Smart Agriculture in Smallholder Farming Systems The experience of the MICCA pilot projects in Kenya and the United Republic of Tanzania. Retrieved from https://www.fao.org/3/i5805e/i5805e.pdf.
- Franzluebbers, A. J., Lemaire, G., de Faccio Carvalho, P. C., Sulc, R. M., & Dedieu, B. (2014). Toward agricultural sustainability through integrated crop-livestock systems: Environmental outcomes. Integrated Crop-Livestock System Impacts on Environmental Processes, 190, 1–3.
- Garrett, R., Ryschawy, J., Bell, L., Cortner, O., Ferreira, J., Garik, A. V., et al. (2020). Drivers of decoupling and recoupling of crop and livestock systems at farm and territorial scales. Ecology and Society, 25(1). Retrieved February 7, 2024, from https://www.ecologyandsociety.org/vol25/iss1/art24/.
- HLPE (2023). Reducing inequalities for food security and nutrition. Rome, CFS HLPE-FSN. Available from https://www.fao.org/cfs/cfs-hlpe/insights/news-insights/news-detail/reducing-inequalities-for-food-security-and-nutrition/en.
- Ignowski, L., Belton, B., Ali, H. et al. (2023). Integrated aquatic and terrestrial food production enhances micronutrient and economic productivity for nutrition-sensitive food systems. Nature Food 4, 866–873
- Integrated Crop-Livestock Systems (ICLS). (n.d.). Retrieved February 7, 2024, from https://www.fao.org/agriculture/crops/thematic-sitemap/theme/spi/scpi-home/managing-ecosystems/integrated-crop-livestock-systems/en/
- Lemaire, G., Franzluebbers, A., Carvalho, P. C. de F., & Dedieu, B. (2014). Integrated crop–livestock systems: Strategies to achieve synergy between agricultural production and environmental quality. Integrated Crop-Livestock System Impacts on Environmental Processes, 190, 4–8.
- Mazzafera, P., Favarin, J. L., & Andrade, S. A. L. D. (2021). Intercropping systems in sustainable agriculture. Frontiers in Sustainable Food Systems, 5, 634361.
- Peterson, C. A., Bell, L. W., Carvalho, P. C. de F., & Gaudin, A. C. M. (2020). Resilience of an Integrated Crop–Livestock System to Climate Change: A Simulation Analysis of Cover Crop Grazing in Southern Brazil. Frontiers in Sustainable Food Systems, 4, 604099.
- Poffenbarger, H., Artz, G., Dahlke, G., Edwards, W., Hanna, M., Russell, J., et al. (2017). An economic analysis of integrated crop-livestock systems in Iowa, U.S.A. Agricultural Systems, 157, 51–69.
- Thornton, P. K., & Herrero, M. (2015). Adapting to climate change in the mixed crop and livestock farming systems in sub-Saharan Africa. Nature Climate Change, 5(9), 830–836.